The National Academy of Engineering (NAE) announced today that Karen Willcox, professor in the Department of Aerospace Engineering and Engineering Mechanics and director of the Oden Institute for Computational Engineering and Sciences and Al Bovik, professor in the Department of Electrical and Computer Engineering at The University of Texas of Austin, have been elected to the prestigious academy for 2022. In addition, alumni Michael Watkins and Ahmad Abdelrazaq have also been elected.
Election to the academy is among the highest professional distinctions bestowed upon an engineer. Membership honors those who have made outstanding contributions to engineering research and practice, including pioneering new and developing fields of technology and making major advancements in the engineering field and profession. In all, 111 new members and 22 foreign members joined the NAE in 2022.
“Throughout their careers, Al, Karen, Michael and Ahmad have been among the nation’s leading experts in their respective fields,” said Roger Bonnecaze, interim dean of the Cockrell School of Engineering. “From space exploration to streaming video quality, these four newly elected members have made significant contributions in a wide variety of engineering disciplines — a testament to the Cockrell School’s breadth, depth and overall excellence. We are extremely proud and honored to call them Texas Engineers.”
During the past decade, more than 15 UT Austin professors have been elected as new members to the NAE, and the university has almost 50 current and retired members.
About the four new members representing UT Austin:
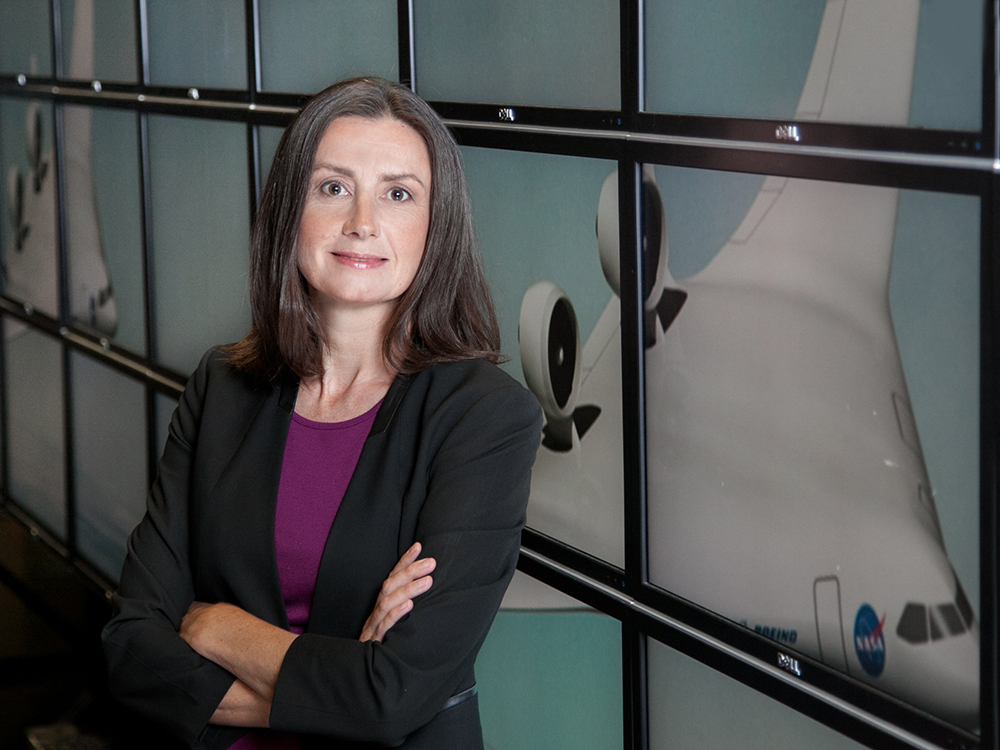
Karen Willcox is director of the Oden Institute for Computational Engineering and Sciences at UT Austin and holds the W.A. “Tex” Moncrief Jr. Endowment in Simulation-Based Engineering and Sciences Chair No. 5 and the Peter O’Donnell Jr. Centennial Chair in Computing Systems. She is also a professor in the Cockrell School’s Department of Aerospace Engineering and Engineering Mechanics. Willcox is being recognized for contributions to computational engineering methods for the design and optimal control of high-dimensional systems with uncertainties. Her research has produced scalable computational methods for design of next-generation engineered systems, with a particular focus on model reduction as a way to learn principled approximations from data and on multifidelity formulations to leverage multiple sources of uncertain information. Prior to joining UT Austin in 2018, Willcox spent 17 years on the faculty at the Massachusetts Institute of Technology, where she served as the founding co-director of the MIT Center for Computational Engineering. She is a fellow of the Society for Industrial and Applied Mathematics and the American Institute of Aeronautics and Astronautics as well as a member of the American Society for Engineering Education. Willcox received a bachelor’s degree in engineering science from the University of Auckland and master’s and doctoral degrees from MIT, both in aeronautics and astronautics.
Michael Watkins (B.S. Aerospace Engineering 1983, M.S. ASE 1985, Ph.D. ASE 1990) is a professor of aerospace and geophysics at the California Institute of Technology, following five years serving as director of NASA’s Jet Propulsion Laboratory. He is being recognized for leadership in the development of space geodesy and leading robotic missions for exploration of the Earth and planetary bodies. Prior to joining JPL as director, Watkins was a professor in the Cockrell School’s Department of Aerospace Engineering and Engineering Mechanics and director of UT’s Center for Space Research. Before that, he spent 22 years at JPL, where he led some of NASA’s most high-profile missions, including the Mars Curiosity Rover and the Cassini, Mars Odyssey and Deep Impact probes, in addition to leading the science development for the GRAIL moon-mapping satellites. Watkins, who is a pioneer in the development and use of gravity data in science applications, also originated the concept for the GRACE and GRACE Follow-On missions, which use twin satellites to measure Earth’s gravity field. Watkins received his bachelor’s, master’s and doctoral degrees from UT Austin, all in aerospace engineering.
Alan Bovik holds the Cockrell Family Regents Chair in Engineering #3 in the Cockrell School of Engineering’s Department of Electrical and Computer Engineering. He is being recognized for contributions to the development of tools for image and video quality assessment. Bovik’s work broadly focuses on creating new theories and algorithms that allow for the perceptually optimized streaming and sharing of visual media. He is the director of the UT Laboratory for Image and Video Engineering and a member of the Wireless Networking and Communication Group, the Institute for Neuroscience and the Machine Learning Laboratory. He has published over 1,000 technical articles and holds several U.S. patents. Bovik, who joined UT Austin in 1984, has received numerous recognitions for his work, including two Emmy Awards, the Progress Medal from the Royal Photographic Society, the Edison Medal from the Institute of Electrical and Electronics Engineers, the IEEE Fourier Award for Signal Processing and the Edwin H. Land Medal from The Optical Society of America. He is a fellow of IEEE, The Optical Society of America, the Society of Photo-Optical and Instrumentation Engineers and honorary fellow of the Royal Photographic Society. Bovik received his bachelor’s, master’s and doctoral degrees from the University of Illinois at Urbana-Champaign, where he has also been honored with a Distinguished Alumni Award.
Ahmad Abdelrazaq (B.S. Civil Engineering 1984, M.S. CE 1986) is senior executive vice president of the Highrise Building and Structural Engineering Divisions at Samsung C&T Corporation. He is being recognized for innovation in design, construction and health monitoring of the world’s tallest and most complex building structures. Since joining Samsung in 2004, Abdelrazaq has been involved in all aspects of construction planning, preconstruction services and structural design of many prominent building projects, including the Burj Khalifa, currently the tallest building in the world, and Jumeriah Gardens in Dubai, and the Samsung HQ, Inchon Tower, Korean World Trade Center and the Yongsan Landmark Tower in Seoul. As chief technical director for the Burj Khalifa project, he played a key role in the development of the structural and foundation systems for the tower from initial design concept through completion. Abdelrazaq also currently serves as a visiting professor at Seoul National University. Previously, he was associate partner and senior project structural engineer at Skidmore Owings & Merrill LLP in Chicago, as well as an adjunct professor at the Illinois Institute of Technology. Abdelrazaq received his bachelor’s and master’s degrees from UT Austin, both in civil engineering.